Chapter 4. Development of methods for the assessment of genotypic diversity in Penicillium
Abstract
Techniques for 1) small-scale isolation of high molecular weight total DNA; 2) heteroduplex mobility assay for screening genotypic diversity; and, 3) identification of polymorphic genetic loci in P. brevicompactum and P. chrysogenum are described.
Isolate selection
Isolates of Penicillium for genetic studies were collected and stored according to the methods presented in Chapter 2. Two species of Penicillium were selected for intensive study based on the strong representation in the culture collection and their good representation across the set of houses studied. Penicillium chrysogenum was selected because this was the most commonly occurring species of the genus in broadloom dust (see Figure 2-4) and was represented by 700 isolates in the accumulated collection. Penicillium brevicompactum was also chosen for further investigation. Although this species was outnumbered in the dataset and culture collection by isolates of P. spinulosum, P. corylophilum, and P. commune, its species circumscription seemed to be more stable and its identification less prone to confusion.
All isolates of P. brevicompactum and P. chrysogenum that had been catalogued during the collection phase of this project were subcultured on several diagnostic media including modified Leonian’s agar (MLA), Czapek’s yeast autolysate agar (CYA) and modified Creatine-sucrose agar (CSA). Cultures were incubated at room temperature and rated for colony characteristics at 1, 2 and 3-week intervals. Microscopic preparations were made after 1 wk from cultures on MLA. Subcultures on MLA were also rated for growth after 1 wk at 5 C and 37 C. Based on the results of these tests, a subset of isolates that demonstrated high phenotypic homogeneity were selected for each species for further study (data not shown).
Isolation of DNA from Penicillium conidia
Many of the existing techniques for isolating fungal DNA were designed for methods requiring milligram amounts of total DNA (Weising et al., 1995). These methods typically involve the preparation of broth cultures, followed by mycelial harvest and grinding. On a small-scale, these procedures are practical; however, the labour-intensive quality of these techniques makes them ill-suited to projects requiring DNA isolations from large numbers of samples. Furthermore, the potential for aerosol generation posed by such large-scale grinding risks potential biological hazards as well as sample cross-contamination. For the purposes of the current project, a modified technique was developed which permits rapid small-scale preparation of total DNA which facilitates the processing of large numbers of samples.
Materials and methods, DNA isolation
Fungal isolates were inoculated centrally on a Petri plate of Weitzman and Silva-Hutner’s agar (WSHA) (Weitzman and Silva Hutner, 1967), and grown for 7 days at room temperature under 12 hr artificial daylight. The plates were flooded with 2 mL of 95 % ethanol and the conidia and mycelium were suspended by gently scraping the surface of the colonies with a sterile bent glass rod. The conidial suspensions were collected in microcentrifuge tubes, centrifuged at 12 Krpm and the supernatant ethanol was discarded. The pellets were dried for 30 min in a vacuum concentrator centrifuge. This protocol yielded approximately 15 mg of pelleted conidia per vial. Each vial was sufficient for a single DNA isolation.
Approximately 15 mg of sterile, acid-cleaned Dicalite 1400 (diatomaceous earth-based swimming pool filter, Grefco Inc., Torrance, California), was added to a tube containing a roughly equal volume of dry, pelleted, ethanol-killed conidia. Following the addition of 10 uL of 70 % EtOH, the mixture was ground with a sterile glass rod for 1 min and suspended in 600 uL of lysis buffer containing 1.4 M NaCl, 2 % w/v CTAB, 200 mM Tris-HCl pH 8.0 and 20 mM EDTA (adapted from Weising et al., 1995). Tubes were incubated at 65 C for 1 hr, during which they were mixed by inversion at 30 min intervals.
After extraction, the tubes were cooled to room temperature and centrifuged at 10 K rpm for 1 min to pellet the Dicalite and cellular debris. The supernatant liquid was extracted twice with chloroform:isoamyl alcohol (24:1) and the DNA was precipitated with 100 % isopropanol for 10 min at -80 C. The pellets were rinsed with 70 % ethanol and dried. The DNA was resuspended in 200 uL Tris-EDTA (10 mM and 1 mM, respectively) (TE) pH 8.0 (Sambrook et al., 1989). Ribonuclease A was added to the DNA at a final concentration of 0.2 ug/uL, and incubated for 30 min at 37 C. The DNA was subsequently extracted with chloroform:isoamyl alcohol as above, and following the addition of sodium acetate to a concentration of 0.3 M, the DNA was precipitated with 250 uL of 100 % ethanol at -80 C. The DNA solution was pelleted and pellets were rinsed with 70 % ethanol, dried and resuspended in 100 uL TE pH 8.0. The concentration of DNA in solution was determined spectrophotometrically.
Results and discussion, DNA isolation
Dicalite was selected as a grinding agent because it is commercially available and inexpensive. It acts both as an abrasive to disrupt conidial walls, and as a bulking agent to ensure surface contact between the abrasive and the biological material. Although tight binding between DNA and glass powder in the presence of high concentrations of chaotropic salts (e.g. sodium iodide) forms the basis of several proprietary technologies for DNA purification (e.g. Geneclean® II, Bio 100 Inc.; Qiagen purification spin-columns), controlled experiments using herring sperm DNA indicated that negligible DNA bound to the Dicalite under the conditions used in this procedure (data not shown). Typically, a single Petri plate of P. brevicompactum grown for 14 days yielded 10 to 100 mg, dry weight, of harvestable conidia. From this, 10 to 100 ug of high molecular weight DNA was isolated.
Many of the currently used methods for the isolation of DNA from harvested cellular material involve grinding procedures that risk generating aerosols. In cases where the fungi submitted to these procedures are either pathogenic, opportunistic or heavily sporulating, a potential biological hazard exists from the generation of viable fungal aerosols. Other risks independent of the viability of conidial or cellular aerosols, such as mycotoxins and allergenic beta-glucans, are greater with large mycelial isolations. In the procedure presented, ethanol serves as the initial harvesting medium. Ethanol is an efficient wetting agent for hydrophobic conidia, which prevents conidial aerosolization during the early stages of preparation. In addition, ethanol treatment kills Penicillium conidia, thereby eliminating the potential of cross culture contamination during the DNA isolation procedures. The small scale of the preparations, and the limited use of grinding due to the inclusion of diatomaceous earth further reduce the potential for the generation of cellular aerosols which could cross-contaminate sample preparations.
Heteroduplex mobility assay (HMA)
This section describes a PCR-based heteroduplex analysis to screen for variability between homologous amplification products from different isolates of P. brevicompactum (Jensen and Straus, 1993). In this method, the PCR-amplified products of a pair of isolates are combined in equal proportion, denatured at 94 C for 3 min and reannealed at 65 C for 5 min. The resulting mixture comprises all possible combinations of compatible DNA strands including two homoduplexes identical to each original amplification product, along with two hybrid DNAs (heteroduplexes) created from the cross-annealing of compatible strands originating from different “parents”. Any sequence differences, including base substitutions, insertions or deletions produce local “bubbles” or “kinks” in the hybrid molecules. These anomalies result in differential electrophoretic migration of these structures relative to duplexed strands 100 % base complimentarity. Thus, small dissimilarities in sequence between two isolates at a given locus can be detected readily.
Materials and methods, HMA
DNA amplification
An identified polymorphic region near the 5′-end of the beta-tubulin gene benA was amplified using primer sequences Bt2a and Bt2b described by Glass and Donaldson (1995). PCRs consisted of 1 unit of Taq DNA polymerase (Boeringher Mannheim), 50 mM KCl, 2.0 mM MgCl2, 250 uM each of dATP, dTTP, dCTP and dGTP, 0.2 mM of each primer and approximately 60 ng high molecular weight template DNA in a total reaction volume of 50 µL overlaid with a drop of sterile mineral oil to prevent evaporation. A template-free reaction was included in each set of reactions. Reactions were carried out in a PTC-100 thermocycler (MJ Research). The typical PCR profile used consisted of 94 C for 30 s to denature, 58 C for 30 s to anneal primers and 72 C for 30 s to extend. This profile was repeated for 30 cycles, followed by a final extension at 72 C for 2 min. PCR yield was roughly quantified based on ethidium bromide staining and UV visualization following electrophoresis on 1.2% agarose gels.
Cloning and sequencing of PCR products
Initial work on the heteroduplex screening method used cloned homologous fragments from a polymorphic region at the 5’ end of the beta tubulin gene, benA. During the initial investigations into the use of the heteroduplex technique, cloned products were used to eliminate the influence of potential PCR artifacts.
Products for cloning were amplified directly from genomic template DNA using 5′-phosphorylated primers. Primers were phosphorylated in 0.2 uL 10 mM ATP, 1 uL 10x polynucleotide kinase buffer (Promega) and 0.5 uL T4 polynucleotide kinase (Promega), 7.3 uL sterile distilled deionized water and 1 uL of 500 uM oligonucleotide primer, incubated at 37 C for 30 min and the enzyme was inactivated by incubation at 65 C for 20 min.
Following amplification and soft gel purification of products (Sambrook et al., 1989), 42 uL aliquots of products were blunt-ended with 5 uL 10x T4 DNA polymerase buffer, 0.25 uL acetylated bovine serum albumin, 100 uM deoxynucleotide triphosphates (dNTPs) and 0.25 uL T4 DNA polymerase (New England Biologicals). The resulting products were extracted once with phenol:chloroform (1:1) and once with chloroform and precipitated at -80 uC by the addition of 0.1 M NaCl and two volumes of cold 100 % ethanol. Prior to ligation, plasmid vector was digested to completion with HincII and treated with shrimp alkaline phosphatase according to suppliers instructions (US Biochemical). Ligation was performed using 45 ng of linearized pUC19 vector, 45 ng insert DNA, 2.4% polyethylene glycol 8000, 2 uL 5x DNA ligase buffer and 0.6 uL DNA ligase (BRL) in a reaction volume made to 10 uL with sterile distilled deionized water and incubated at room temperature for 3-4 hr. Following ligation, reactions were diluted to 40 uL total volume with 1x DNA ligase buffer and transformed into E. coli strains DH5-alpha and JM109 using the method described by Hanahan (1985). Cells were plated in 3 replicates of 100 uL aliquots of transformation reaction onto LB agar containing 60 ug/mL ampicillin. Isopropylthio-alpha-D-galactoside (IPTG) (80 ug/mL) and 5-bromo-4-chloro-3-indolyl-alpha-D-galactoside (XGAL) (20 ng/mL) were incorporated to permit blue/white selection. Recombinant plasmids were isolated by alkaline lysis (Sambrook et al., 1989). Cloned DNA fragments were sequenced by the method of Sanger and co-workers (1977), using universal and reverse primers for pUC19.
Preparation and analysis of DNA heteroduplexes
Preliminary experiments usied cloned, sequenced DNA fragments which were PCR-amplified as described above using the Bt2 primer set. Products were amplified directly from total DNA. For each locus examined, PCRs were diluted to 50 % of the original concentration, with 4 mM EDTA and 50 mM KCl and combined in equimolar proportion in a total volume of 10 uL, and overlaid with a drop of sterile mineral oil. PCRs of sequentially numbered isolates were combined in an pair-wise manner such that each numerically adjacent pair were combined with each-other, and the first and last isolate of each series were combined. Reactions were heated to boiling for 4 min and immediately annealed at 65 C for 6 min. Based upon the results of this first pass, all adjacent “like” isolates were reduced to a single representative strain by transitive property. Subsequent rounds of HMA compared non- numerically juxtaposed “proxy” isolates ultimately reducing the entire population to a set of genotypically distinct alleles represented by a minimum number of proxy isolates for each locus tested.
Electrophoresis and imaging
Prior to use in heteroduplexing reactions, the quality and yield of PCRs was assessed by electrophoresis on 1.5 % agarose gels in 1 x TAE. Agarose and proprietary gels were stained in 250 ng/mL ethidium bromide for 5-30 min and destained in distilled water for 10-30 min. Polyacrylamide gels were stained in 250 ng/mL ethidium bromide for 2-4 hr and destained in distilled water for 2 hr to overnight. All gels were visualized on an ultraviolet light transilluminator at 300 nm (Fotodyne).
Resolution of heteroduplexed DNAs on Phast system
Initial experiments to resolve heteroduplexed DNAs were conducted using the Phast gel electrophoresis system (Pharmacia, New Jersey). The Phast gel depicted in Figure 4-1 shows slow-migrating heteroduplexed DNA bands in lanes 2-5 and 7-8. The homoduplex band front is shown by the migration of native PCR product in lane 6. Despite that the Phast system provided good resolution of heteroduplexed DNAs, this system was not amenable to scale-up use given the 12 lane capacity of Phast gels.
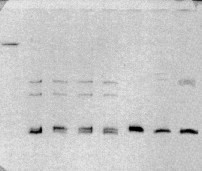
Figure 4-1: Demonstration of DNA heteroduplexes using the Phast gel system. Lanes contain (from left to right) 1) 1 ug HindIII-digested λ-DNA; lanes 2-8 contain heteroduplexed PCR products for the following pairs of P. brevicompactum isolates: 2) B91 + B119; 3) B119 + B132; 4) B 132 + B166; 5) B166 + ALG1; 6) ALG1 + DAOM 214776; 7) DAOM 214776 + DAOM 214783 (deposited as P. chrysogenum, but appears to be misidentified); and 8) DAOM 214783 + B36.
Horizontal gel electrophoresis (HGE) in HMA
A technique of horizontal gel electrophoresis (HGE) was investigated for use in resolving heteroduplexed DNAs. In this method, agarose gel casting trays (BioRad minisubmarine) were modified by gluing a spacer strip of 6 mm square extruded poly(methyl-methacrylate) (PMMA) rod (General Electric), equal in length to the casting tray, into each corner of the tray using a glue that consisted of 1 % (w/v) PMMA and 1 % (v/v) acetic acid in dichloromethane. The volume of the gel tray was recalculated based on the decreased width. A removable cover plate designed to rest on top of the square rods was cut from 1/8″ thick PMMA (Plexiglass, General Electric). The cover plate was cut to approximately 1 cm shorter than the total length of the gel tray to accommodate insertion of the comb. The use of a cover plate ensured a uniform thickness for the gel. In addition, the cover plate prevented contact with air during polymerization. The ends of the tray were sealed with masking tape (3M). The cover plate and comb were put in place prior to pouring. A solution of 7.7 % acrylamide, 0.3 % bisacrylamide and 0.04 % ammonium persulfate was prepared in 1 x TAE (Sambrook et al., 1989), to which 0.2 % N,N,N’,N’-tetramethylethylenediamine (TEMED) was added immediately prior to casting after degassing of the solution.
Preliminary experiments using this method were conducted in which running buffer was in contact with the upper surface of the gel. In this configuration, it was found that a vertical ionic gradient was established during electrophoresis. This ionic gradient caused the differential migration of the lower portion of bands relative to the upper portion, producing an appearance of bending in cross-section (Figure 4-2). Visualization of these gels in face view showed smeared bands (not shown).
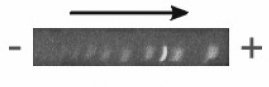
Figure 4-2: Cross-section of band fronts of Promega 100 bp marker run on horizontal PAG cast and run without top-plate. Arrow indicates direction of electrophoretic migration. Note the convex configuration of band fronts in direction of anode (+). This bent morphology produced considerable blurring of bands in face view (not shown).
To address this problem, a cover plate was kept in position on the upper surface of the gel during electrophoresis to prevent contact with the running buffer. Exclusion of the running buffer from contacting the upper surface of the gel prevented ionic migration across the gel-buffer interface, thus preventing the formation of a vertical, ionic gradient in the gel matrix. This modification tended to sharpen the appearance of bands greatly.
Comparative sets of samples were electrophoresed in 1.5 % agarose (BioRad) as well as the proprietary media, Clearose®, a modified agarose (Elchrom), and 9 % Poly-NAT® (poly N-acryloyltris-(hydroxymethylaminomethane, Elchrom). Proprietary gel media electrophoresed in 0.75 x TAE (30 mM tris-acetate, 0.75 mM EDTA adjusted to pH 8.0) (Sambrook, 1989) using a temperature controlled, buffer-recirculating electrophoresis system (SEA 2000, Elchrom) running at 40 C with a field strength of 5 Vcm-1. Polyacrylamide and poly-NAT gels were stained for 1 hr in 250 ng/mL ethidium bromide and destained in distilled water for 3-4 hr. Concentrations of amplification products were standardized based on band intensity as compared to a quantitative standard.
Results and discussion, HGE
Preliminary investigations for appropriate electrophoresis methods used a set of morphologically and physiologically indistinguishable isolates of P. brevicompactum, notably three isolates, 114, 132 and 244 which were shown to differ in the Bt2 locus by sequencing on cloned PCR-amplified products (Figure 4-3). Different allelic forms of Bt2 were observed for each of these isolates using heteroduplex techniques. An additional isolate, 112, which did not form a heteroduplex with 114, was selected as a control. Aligned sequences of the cloned fragments for the Bt2 locus of isolates 114, 132 and 244 are shown in Appendix F-1. Isolates 114 and 244 have the greatest sequence similarity (15 base substitutions and two non-contiguous insertions/ deletions). Isolates 114 and 132 differ by 42 base substitutions, 9 non-contiguous insertions/deletions and a four-base contiguous insertion/deletion. Isolates 132 and 244 differ by 44 base substitutions, 8 non-contiguous insertions/deletions, and the same four-base contiguous insertion/deletion. Sequence obtained for the Bt2 amplicon from isolate 112 was identical to that of isolate 114 (data not shown).
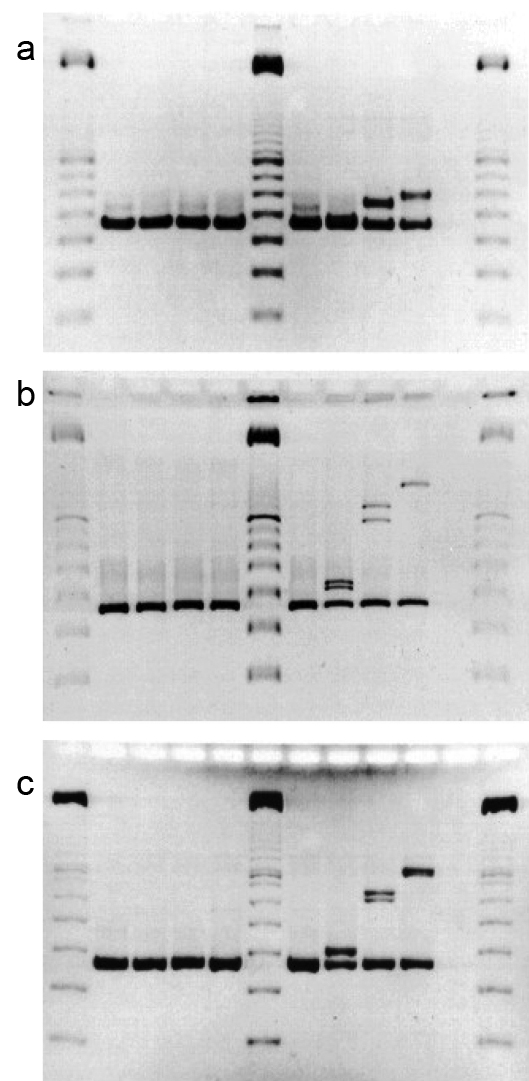
Figure 4-3: Comparison of media in HGE for the resolution of DNA HDXs. Gel a) shows a Clearose BG © gel run in 0.75 x TAE at 40 °C for 60 min under a field strength of 5 V/cm; Gel b) shows a 9% poly-NAT gel run as above for 225 min; Gel c) shows an 8% acrylamide, 0.2 % bisacrylamide gel run as above for 75 min. Lanes of all gels contain cloned PCR products of Bt2 primer pair (from left to right) 1, 6 and 12) Promega 100 bp marker; 2) B112; 3) B114; 4) B132.1; 5) B244. Lanes 7-10 contained heteroduplexed cloned products in the following combinations; 7) B112 + B114; 8) B114 + B244; 9) B114 + B132.1; 10) B132.1 + B244.
Each of these clones was amplified by PCR and heteroduplexed as described in Materials and Methods. The resulting reassociated structures were compared under three different conditions of electrophoresis (Figure 4-3). These conditions included Clearose BG (Elchrom) (Figure 4-3a), 9 % poly-NAT (Elchrom) (Figure 4-3b) and low-crosslinked 8 % polyacrylamide (crosslinked with 0.2 % bisacrylamide) (Fig. 4-3c). These gels show that the low-crosslinked polyacrylamide provided the greatest level discriminate of heteroduplexed structures. Of the pre-cast gels, 9 % poly-NAT provide an acceptable degree of resolution for situations where the volume of analyses precludes in-house gel preparation. All of these analyses were completed on a temperature-regulated horizontal gel apparatus that is amenable to polyacrylamide or other polymeric gel substrates (SEA 2000, Elchrom). Horizontal gel electrophoresis system gels have the advantages of rapid loading, and easy gel casting and manipulation inherent to this configuration (Bellomy and Record, 1989).
The heteroduplex patterns that appeared in the poly-NAT and polyacrylamide electrophoretic gels (Figs. 4-3b &-c, respectively) corresponded to the mobilities expected based on the differences in sequence of the fragments such that the order of retardation of heteroduplex mobility corresponds to the degree of sequence dissimilarity. However, as pointed out by White et al. (1992) and Ganguly et al. (1993), the actual gel mobility retardation in heteroduplex structures is related in a complex manner both to the type and distribution of sequence differences. Clustered base sequence changes that are centrally located produce local destabilization domains and have a much larger effect on mobility than non-contiguous differences dispersed throughout the molecule. However, the most pronounced heteroduplex mobility shifts result from molecular kinks caused by insertions or deletions between paired strands (Ganguly et al., 1993; Wang et al., 1992). This is seen in the substantial difference between the heteroduplex mobilities of the 114/132 and 132/244 heteroduplexes. Although the structures produced from 114/132 and 132/244 pairings show substantial difference in electrophoretic mobilities, the cumulative dissimilarities are only 55 and 56, respectively. The differences between these mobilities relative to both the homoduplexed DNAs and the 114/244 heteroduplexes seem unduly large because this total cumulative sequence difference between the 114/132 and 132/244 heteroduplexes is only one additional single base insertion/deletion in the latter.
Vertical gel electrophoresis (VGE) in HMA
The low level of sequence variation between PCR fragments necessitated a greater running distance to achieve adequate resolution of DNA heteroduplexes. To address this need, the method of vertical gel electrophoresis (VGE) described by Xing and colleagues (1996) was used, in which a hybrid low crosslinked polyacrylamide / agarose gel was cast between large glass plates using a vertically-oriented protein-type electrophoresis apparatus (e.g. Protean II, BioRad, La Jolla, CA). The gel matrix was made to be weakly denaturing by the addition of glycerol prior to casting. This weakly denaturing gel matrix destabilized small heteroduplexes and facilitated their detection. This system was used for scaled-up evaluation of PCR-amplified polymorphic loci from numerous fungal isolates since the system permitted the simultaneous electrophoresis of 100 products per run.
Similar to the horizontal method, a solution of 12.0 % acrylamide, 0.2 % bisacrylamide and 0.04 % ammonium persulfate was prepared in 1 x TBE to which 1.2 % agarose and 0.2 % N,N,N’,N’-tetramethyl-ethylenediamine (TEMED) were added after degassing of the solution and immediately prior to casting. Gels were cast in a BioRad Protean electrophoresis apparatus at 1 mm thick, and allowed to polymerise for 4-5 hours prior to use. For electrophoresis, gels were cooled to 12 °C using a constant temperature refrigerated water bath and electrophoresed at 10 V/cm for up to 20 hr. Following electrophoresis, gels were stained in 250 ng/mL ethidium bromide for 2 hr, destained in distilled water for 3-4 hr prior to imaging. The large size of the gels and their relative fragility made their handling difficult. Custom plexiglass staining trays were constructed to accommodate these large gels. The trays were assembled using walls of PMMA plexiglass (General Electric) glued with 1 % (w/v) PMMA and 1 % (v/v) acetic acid in dichloromethane to a bottom plate of Acrylite OP-4 (General Electric), an ultraviolet transparent plastic sheet. The use of these trays allowed the gels to be stained, destained and visualized in a manner that limited their direct handling.
HMA Screening approaches
For scale-up comparison of PCR products, two general screening approaches were used.
Overlapped pairs
In the first method, all isolates were screened in overlapping pairs. In this technique, PCR products of all isolates were arranged in fixed, arbitrary sequence. For the initial round of HMA, all adjacent pairs were compared. For example, the hypothetical set A, B, C & D, would be compared in the pairs A+B, B+C, C+D and D+A. Following this initial round of HMA, all like-isolates were collapsed to a single representative by transitive property. A smaller second round of HMA compared alternating combinations of the remaining representative isolates. Often, with four rounds of reductive HMA, it was possible to determine haplotype for the entire set of 198 isolates of P. chrysogenum and 75 isolates of P. brevicompactum. An example of this approach is shown in Figure 4-4.
Universal heteroduplex generator
A second screening approach used for HMA involved the selection of a single isolate as a “universal heteroduplex generator”. This isolate was selected on the basis of it being relatively distant from the panel of test isolates, typically from a closely related species. For example, P. polonicum was used as a universal heteroduplex generator for P. chrysogenum test panel. In this approach, each isolate was paired with the universal heteroduplex generator and submitted to electrophoresis (as above) under identical conditions. An example gel run using this approach is shown in Figure 4-5.
Results and discussion, VGE
Overall, the use of the vertical electrophoresis method increased the resolution of small differences by HMA due to the greater length of the gels. As well, the larger format of the BioRad system allowed the electrophoresis of 25 lanes per gel. Gels were run back-to-back in the configuration recommended by the manufacturer. Thus, the operation of two electrophoresis apparatuses in this configuration permitted the simultaneous screening of 100 isolates.
Figure 4-4
Figure 4-5
The overlapping pairs screening method was used to assess haplotype for all loci in both P. brevicompactum and P. chrysogenum. This technique performed well for both test panels which in both cases were dominated by a single genotype. Using this method it was possible to reduce the test panel by an average of approximately 80 % in all cases following the first round of HMA. This screening approach was also convenient since it did not require the inclusion of external lane standards to account for slight variation in electrophoretic conditions or the separation of gel runs in time. Rather, each lane compared two contiguously accessioned isolates directly, eliminating the need for relative assessment of band migration in different lanes. Figure 4-4 shows a gel in which P. chrysogenum isolates were compared at the Bt2 locus employing the overlapping pairs screening approach. Note that heteroduplex bands are absent in lanes 1-6, 9-12, 15-17, 20 and 23-25, indicating that these lanes contain isolate pairs with indistinguishable sequences at this locus. In contrast, the isolate common to lanes 7-8, 18-19 and 21-22 differs from its paired isolates in these two lanes by a total of 4 non-contiguous base substitutions at positions 127 (A/G), 267 (A/G) and 351 (T/C) (total product length of 433 bp) and a deletion of 2 contiguous bases at position 242-243 (see Appendix G). The small heteroduplex shift indicated by arrows in lanes 13-14 differed from its paired isolates in these two lanes by a total of 7 non-contiguous base substitutions at positions 134 (C/T), 195 (G/A), 198 (T/C), 233 (A/G), 246 (G/A), 267 (A/G) and 351 (T/C). The smallest difference revealed using this technique was a single base substitution between isolates C8.12 and C8.24 at position 363 (T/G) in the 548 bp ITS fragment amplified from P. chrysogenum isolates (gel not shown) (see Appendix G).
For loci that demonstrated greater frequency of allelic differences, a screening method employing a universal heteroduplex generator method was used in addition to the overlapping pairs method. The use of a divergent universal heteroduplex generator increased the separation between heteroduplex and homoduplex band fronts, reducing interference of the latter in evaluating band shifts. Figure 4-5 shows an HDX gel of P. brevicompactum using the Bt2 locus using a universal heteroduplex generator (in this case the same locus amplified from a distant isolate of P. brevicompactum, B91 (=B132.1, see Chapter 5, Table 5-3)). A small shift in mobility can be seen in the heteroduplex bands in lanes 3 and 19, relative to lanes 2, 4, 6, 7, 10-12, 15-18 and 20-24. This shift between these two groups was correlated to a difference of 4 non-contiguous base substitutions at positions 55 (G/A), 75 (A/T), 258 (T/G) and 420 (G/A), along with a single, contiguous 2-base substitution at position 71-71 (AT/GC) in a total product length of 438 bp (see Appendix F). Similarly, a larger shift between the latter group and lanes 5, 8 and 9 was correlated to 5 non-contiguous base substitutions at positions 60 (T/A), 148 (C/T), 158 (T/G), 259 (G/T) and 420 (A/G), with a contiguous 2-base substitution at position 74-75 (CT/TA) in a product of 438 bp in length. Lanes 13 an 14 contained control of isolate B91.
Identification of polymorphic genetic loci
Preliminary investigation to determine polymorphic loci for use in the characterization of isolates of P. brevicompactum and P. chrysogenum used a randomly selected test panel of 10 isolates for each of these species. Primer pairs that amplified intron regions in conserved metabolic and structural proteins as well as the internal transcribed spacer regions (ITS1-2) of nuclear ribosomal DNA were assessed for their ability to discriminate strains using HMA. Tables 4-1 and 4-2 summarize the loci investigated for the screening of P. brevicompactum and P. chrysogenum isolates, respectively. For P. brevicompactum 7 loci were investigated, three of which, benA, his4 and ITS, showed readily observable polymorphisms with HMA (see Table 4-1). Similarly, four informative loci (acuA, benA, ITS and trxB) were identified from preliminary studies of a P. chrysogenum test panel that investigated 13 candidate loci (see Table 4-2).
Phylogenetic analysis of Penicillium sensu stricto
An analysis of phylogeny within the genus Penicillium was conducted in an effort to determine 1) the placement of P. brevicompactum and P. chrysogenum within the genus, and 2) to assist in the selection of appropriate outgroup taxa for later use in this study. Subgenus Biverticillium was not included in the present analysis because this group consists of anamorphs of the genus Talaromyces, which is only distantly related to Eupenicillium (Lobuglio et al., 1993).
Sequence data for the internal transcribed spacer and partial 28S regions of nuclear ribosomal DNA collected by Dr. Steve Peterson (United States Department of Agriculture, Northern Regional Research Laboratories in Peoria, Illinois) were obtained from GenBank for a set of 85 species of Penicillium including representatives of all accepted subgenera comprising the genus in the strict sense (e.g. subgen. Aspergilloides, Exilicaulis, Divaricatum, Furcatum and Penicillium) (see Table 4-3). A similar analysis of this dataset by parsimony methods is presented by Peterson (2000). Most of these sequences originated from authentic isolates from the NRRL culture collection. Sequences were aligned using Clustal X (version 1.8) (see Jeannmougin et al., 1998; Thompson et al., 1997) and a distance tree was generated using Neighbor-Joining. Alignment of the dataset is provided in Appendix D. Tree branch support was determined by 1000 bootstrap replications. Trees were formatted using version 1.5.2 of TreeView for Microsoft Windows 32 bit (Roderick Page, Oxford University), and formatted using Corel Draw version 8.232 (Corel Corporation, Ottawa). Penicilliopsis clavariiformis was used as an outgroup taxon. The absence of partial 28S sequence prohibited the inclusion of P. brevicompactum in this analysis.
Table 4-1 Candidate loci examined for polymorphisms in P. brevicompactum | |||
Gene |
Source of primers |
No. primer pairs tested |
# with polymorphisms |
anonymous locus (A1) | Glass & Donaldson, 1995 |
1 |
|
Beta-tubulin (benA) | Glass & Donaldson, 1995 |
2 |
1 |
Histone 3 (his3) | Glass & Donaldson, 1995 |
1 |
|
Histone 4 (his4) | Glass & Donaldson, 1995 |
1 |
1 |
Phosphoglycerate kinase (pgk) | Glass & Donaldson, 1995 |
1 |
|
rDNA Intergenic spacer region (IGS) | this study* |
2 |
|
rDNA Internal transcribed spacer region (ITS1-5.8S-ITS2) | White et al., 1989 |
1 |
1 |
* Primer pairs developed in this study were based on gene sequences available in GenBank |
Table 4-2 Candidate loci examined for polymorphisms in P. chrysogenum | |||
Gene | Source of primers |
No. primer pairs tested |
# with polymorphisms |
Acid phosphatase (phoG) | this study* |
1 |
|
Antifungal protein PAF (paf) | this study |
1 |
|
Acetyl co-enzyme A synthase (acuA) | this study |
2 |
2 |
Beta-tubulin (benA) | Glass & Donaldson, 1995 |
2 |
1 |
Histone 3 (his3) | Glass & Donaldson, 1995 |
1 |
|
Histone 4 (his4) | Glass & Donaldson, 1995 |
1 |
|
Nitrogen regulation protein (areA) | this study |
1 |
|
Orotidine-5′-phosphate decarboxylase (pyrG) | this study |
1 |
|
Phosphoglycerate kinase (pgk) | Glass & Donaldson, 1995 & this study |
2 |
|
rDNA Intergenic spacer region (IGS) | this study |
2 |
|
rDNA Internal transcribed spacer region (ITS1-5.8S-ITS2) | White et al., 1989 |
1 |
1 |
Thioredoxin reductase (trxB) | this study |
1 |
1 |
Xylanase (xyl) | this study |
3 |
|
* Primer pairs developed in this study were based on gene sequences from P. chrysogenum deposited in GenBank |
Table 4-3 Sources of nuclear ribosomal ITS and partial 28S sequences used in this study | |||
Identification | Strains number | Status | GenBank accession |
Eupenicillium cinnamopurpureum DB Scott & Stolk | NRRL 3326 | ex-type | AF033414 |
Eu. crustaceum F Ludw. | NRRL 3332 | ex-type | AF033466 |
Eu. egyptiacum Beyma | NRRL 2090 | ex-type | AF033467 |
Eu. lapidosum DB Scott & Stolk | NRRL 718 | ex-type | AF033409 |
Eu. shearii Stolk & DB Scott | NRRL 715 | ex-type | AF033420 |
Hemicarpenteles paradoxus AK Sarbhoy & Elphick | NRRL 2162 | ex-type | AF033484 |
Penicilliopsis clavariiformis Solms | NRRL 2482 | ex-type | AF033391 |
Penicillium adametzii Zalessky | NRRL 736 | AF034459 | |
P. adametzii Zalessky | NRRL 737 | ex-type | AF033401 |
P. adametzioides* Abe ex G Smith | NRRL 3405 | ex-type | AF033403 |
P. asperosporum G Smith | NRRL 3411 | ex-type | AF033412 |
P. atramentosum Thom | NRRL 795 | ex-type | AF033483 |
P. atrovenetum G Smith | NRRL 2571 | ex-type | AF033492 |
P. aurantiogriseum Dierckx | NRRL 971 | ex-type | AF033476 |
P. bilaiae Chalab. | NRRL 3391 | ex-type | AF033402 |
P. camemberti Thom | NRRL 874 | ex-type | AF034453 |
P. camemberti Thom | NRRL 875 | AF033474 | |
P. canescens Sopp | NRRL 2147 | AF034463 | |
P. canescens Sopp | NRRL 910 | ex-type | AF033493 |
P. charlesii* G Smith | NRRL 778 | ex-type | AF033400 |
P. chermesinum Biourge | NRRL 735 | ex-type | AF033413 |
P. chrysogenum Thom | NRRL 807 | ex-type | AF033465 |
P. chrysogenum Thom | NRRL 824 | Fleming | AF034450 |
P. cinerascens Biourge | NRRL 748 | AF033455 | |
P. citreonigrum Dierckx | NRRL 761 | ex-type | AF033456 |
P. citrinum Thom | NRRL 1841 | ex-type | AF033422 |
P. coprophilum* (Berk. & Curtis) Siefert & Samson | NRRL 13627 | ex-type | AF033469 |
P. corylophilum (Berk. & Curtis) Siefert & Samson | NRRL 793 | AF034456 | |
P. corylophilum (Berk. & Curtis) Siefert & Samson | NRRL 802 | ex-type | AF033450 |
P. corylophilum (Berk. & Curtis) Siefert & Samson | NRRL 803 | AF034457 | |
P. crustosum Thom | NRRL 968 | AF033472 | |
P. daleae Zalessky | NRRL 922 | ex-type | AF033442 |
P. decumbens Thom | NRRL 741 | ex-type | AF033453 |
P. decumbens Thom | NRRL 742 | AF034458 | |
P. digitatum (Pers.:Fr.) Sacc. | NRRL 786 | ex-type | AF033471 |
P. donkii Stolk | NRRL 5562 | ex-type | AF033445 |
P. echinulatum Raper & Thom | NRRL 1151 | ex-type | AF033473 |
P. expansum Link | NRRL 974 | AF033479 | |
P. fellutanum Biourge | NRRL 746 | ex-type | AF033399 |
P. fuscum* (Sopp) Biourge | NRRL 721 | ex-type | AF033443 |
P. glabrum (Wehmer) Westling | NRRL 766 | ex-type | AF033407 |
P. griseofulvum Dierckx | NRRL 2300 | ex-type | AF033468 |
P. griseofulvum Dierckx | NRRL 734 | AF034452 | |
P. griseoroseum | NRRL 820 | AF034857 | |
P. griseoroseum | NRRL 832 | AF034449 | |
P. herquei Bainier & Sartory | NRRL 1040 | ex-type | AF033405 |
P. janthinellum Biourge | NRRL 2016 | ex-type | AF033434 |
P. kojigenum* G Smith | NRRL 3442 | ex-type | AF033489 |
P. lividum Westling | NRRL 754 | ex-type | AF033406 |
P. melinii Thom | NRRL 2041 | ex-type | AF033449 |
P. miczynskii Zalessky | NRRL 1077 | ex-type | AF033416 |
P. notatum* Westling | NRRL 821 | ex-type | AF034451 |
P. ochrochloron Biourge | NRRL 926 | ex-type | AF178516 |
P. oxalicum Currie & Thom | NRRL 787 | ex-type | AF033438 |
P. oxalicum Currie & Thom | NRRL 790 | AF034455 | |
P. paxilli Bainier | NRRL 2008 | ex-type | AF033426 |
P. polonicum* Zalessky | NRRL 995 | ex-type | AF033475 |
P. purpurescens (Sopp) Biourge | NRRL 720 | ex-type | AF033408 |
P. raciborskii* Zalessky | NRRL 2150 | ex-type | AF033447 |
P. raistrickii G Smith | NRRL 2039 | ex-type | AF033491 |
P. raperi G Smith | NRRL 2674 | ex-type | AF033433 |
P. restrictum Gilman & Abbott | NRRL 1748 | ex-type | AF033457 |
P. restrictum Gilman & Abbott | NRRL 25744 | AF033459 | |
P. rolfsii Thom | NRRL 1078 | ex-type | AF033439 |
P. roseopurpureum* Dierckx | NRRL 2064 | ex-type | AF033415 |
P. roseopurpureum* Dierckx | NRRL 733 | AF034462 | |
P. sartoryi Thom | NRRL 783 | ex-type | AF033421 |
P. sclerotigenum W Yamam. | NRRL 3461 | AF033470 | |
P. sclerotiorum Beyma | NRRL 2074 | ex-type | AF033404 |
P. simplicissimum HZ Kong & ZT Qi | NRRL 1075 | ex-type | AF033440 |
P. soppii Zalessky | NRRL 2023 | ex-type | AF033488 |
P. spinulosum Thom | NRRL 1750 | ex-type | AF033410 |
P. spinulosum Thom | NRRL 728 | AF034461 | |
P. sumatrense* v. Szilvinyi | NRRL 779 | ex-type | AF033424 |
P. swiecickii* Zalessky | NRRL 918 | ex-type | AF033490 |
P. thomii Maire | NRRL 2077 | ex-type | AF034448 |
P. thomii Maire | NRRL 760 | AF034460 | |
P. turbatum Westling | NRRL 757 | ex-type | AF034454 |
P. turbatum Westling | NRRL 759 | AF033452 | |
P. velutinum Beyma | NRRL 2069 | ex-type | AF033448 |
P. vinaceum Gilman & Abbott | NRRL 739 | ex-type | AF033461 |
P. viridicatum Westling | NRRL 958 | ex-type | AF033477 |
P. viridicatum Westling | NRRL 961 | AF033478 | |
P. waksmanii Zalessky | NRRL 777 | ex-type | AF033417 |
P. westlingii Zalessky | NRRL 800 | ex-type | AF033423 |
A Neighbor-Joining tree rooted using Penicilliopsis clavariiformis is shown in Figure 4-6. An unrooted version of this tree is presented for comparison in Figure 4-7. Branches for which bootstrap values were greater than 50 % are indicated as thick lines. Bootstrap support at greater than 80 % is indicated by an asterisk. Ex-type strains are indicated by “[T]”, and subgeneric classification following Pitt (1979) is indicated by color-coded branches.
The terverticillate taxa classified in Penicillium subgen. Penicillium formed a well-supported monophyletic clade at the top of the tree (indicated in red). Penicillium chrysogenum isolates grouped centrally within this clade, and were sister to P. griseofulvum. Isolates of Penicillium subgen. Aspergilloides formed a clade (bootstrap support less than 50 %) that was sister to the subgenus Penicillium clade. Several extraneous taxa, including P. adametzii, P. herquei and P. asperosporum (subgen. Furcatum sensu Pitt, 1980) were also found within this group. Representatives of subgenus Furcatum (indicated in yellow) were interspersed throughout the tree, indicating the multiple origin of the furcate conidiophore form characteristic of this group. Similarly, isolates of subgenus Divaricatum (indicated in blue) appeared throughout the tree. Divaricate conidiophore morphology appears to be the ancestral branching state, as it forms the backbone of the phylogeny. A multiple origin for the furcate form and an ancestral divaricate morphology for the genus provide the best interpretation of the internal branching topology of this tree. The principal clades identified in this analysis corresponded well to those observed by Peterson (2000) in a parsimony-based analysis of this dataset. The numbered groups observed by Peterson (2000) are indicated on the dendrogram in Figure 4-6 on the corresponding branches. Peterson’s results concur that penicillius complexity is not a reliable phylogenetic predictor, and that this feature is likely influenced by aspects of life history and dispersal of the species (or lineage) in question. The exception is the terverticillate Penicillia, which in both analyses formed a well-supported monophyletic group. Within this group, however, species were poorly separated. The employment of faster-evolving genes may better resolve these taxa.
Penicillium chrysogenum
A subset of the above dataset limited to ITS1-5.8S-ITS2 region of GenBank sequences of 36 taxa in Penicillium sensu stricto collected by Dr. Steve Peterson (USDA, Peoria) were reanalyzed as above. Other sequences included in this dataset were representatives of Wallaceburg isolates of P. brevicompactum, P. chrysogenum and P. polonicum, as well as ex-type strains of species considered to be closely related to these taxa (e.g. P. aethiopicum CBS 484.84; P. olsonii CBS 232.32, P. stoloniferum ATCC 10111 and P. brevicompactum DAOM 193712). Sequences were analyzed and a distance tree was generated, as described above. The Neighbor-Joining tree shown in Figure 4-8 was rooted using Eupenicillium lapidosum and Penicillium biliae (subgenus Aspergilloides). Isolates representing the P. chrysogenum complex group together in a weakly supported clade centrally within subgenus Penicillium (indicated as clade a, Figure 4-8). Within this group, two voucher strains deposited as P. notatum (NRRL 824 from Fleming and NRRL 832 from Biourge), the Wallaceburg strains represented by C8.12 (179 isolates in total) and P. nalgiovense (ex-type strain NRRL 911) formed a well-supported clade.
Penicillium brevicompactum
Isolates of P. brevicompactum formed a well-supported clade sister to the terverticillates (indicated as clade b, Figure 4-8). Within the P. brevicompactum clade, two well-defined groups were observed. The first of these contained the ex-type strains of P. brevicompactum (DAOM 193712) and P. stoloniferum (ATCC 10111) along with 60 isolates from the Wallaceburg collection represented by B65.4 (46 isolates) and B251 (15 isolate). The second group consisted of 10 Wallaceburg isolates represented by B132.1.
Figure 4-8